News
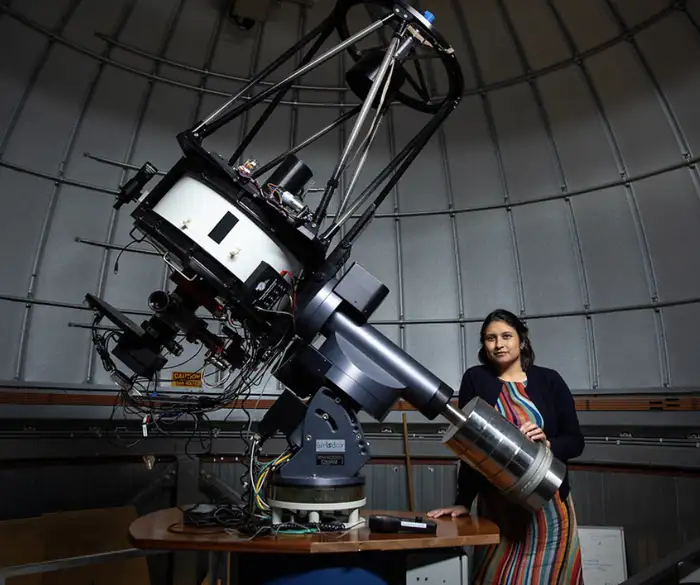
Featured
Samaya Nissanke receives AAS HEAD 2024 Mid-Career Prize
2024 Mid-Career Prize (High Energy Astrophysics Division) of AAS for astrophysicist Samaya Nissanke, for the development of novel techniques to extract fundamental physics from astronomical data, paving the way for the era of GW multi-messenger astronomy.
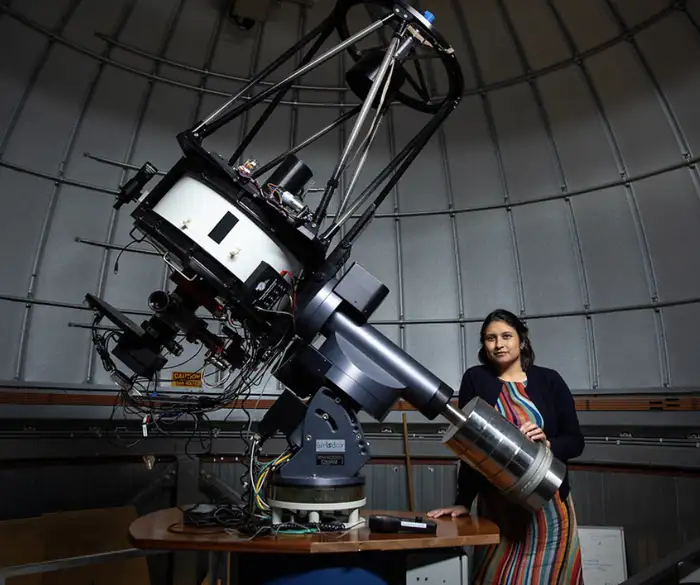
Samaya Nissanke receives AAS HEAD 2024 Mid-Career Prize
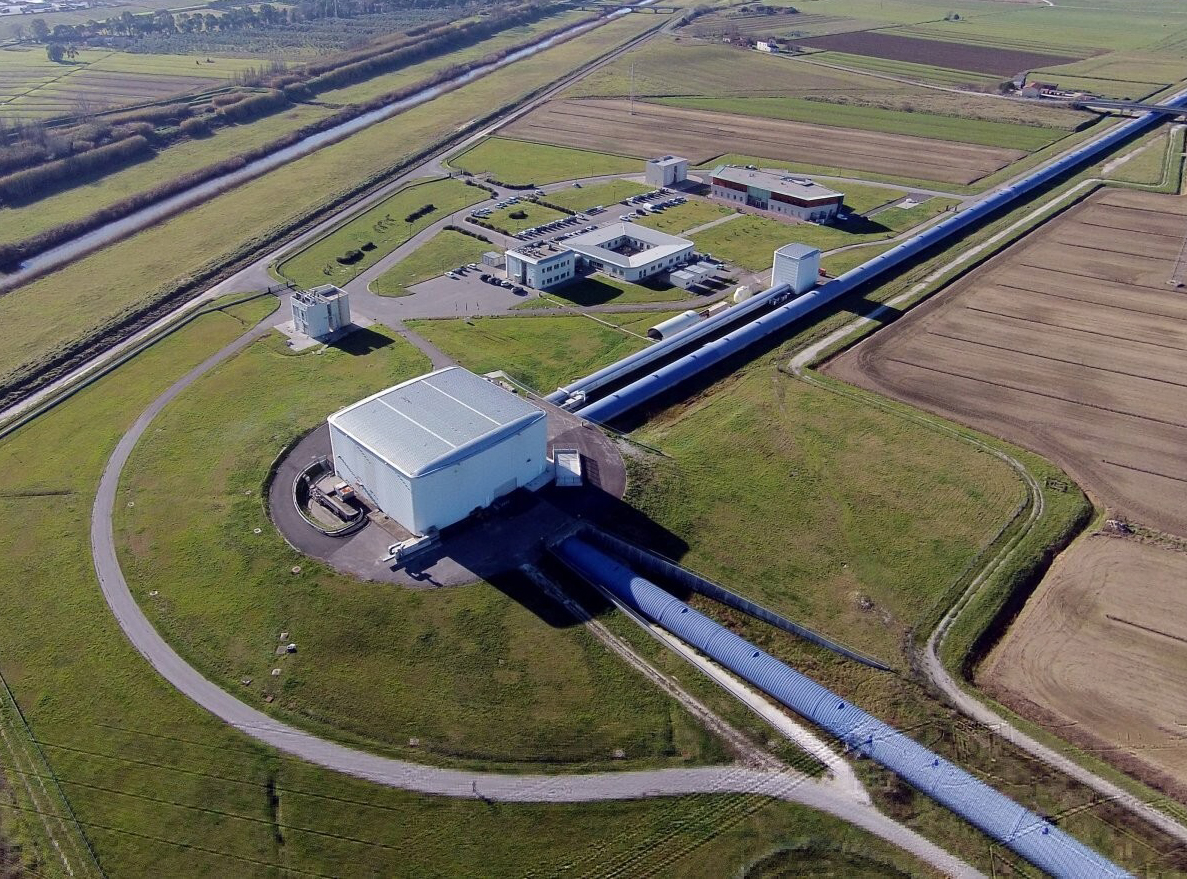
NWO funding for further improvements to Virgo gravitational wave detector
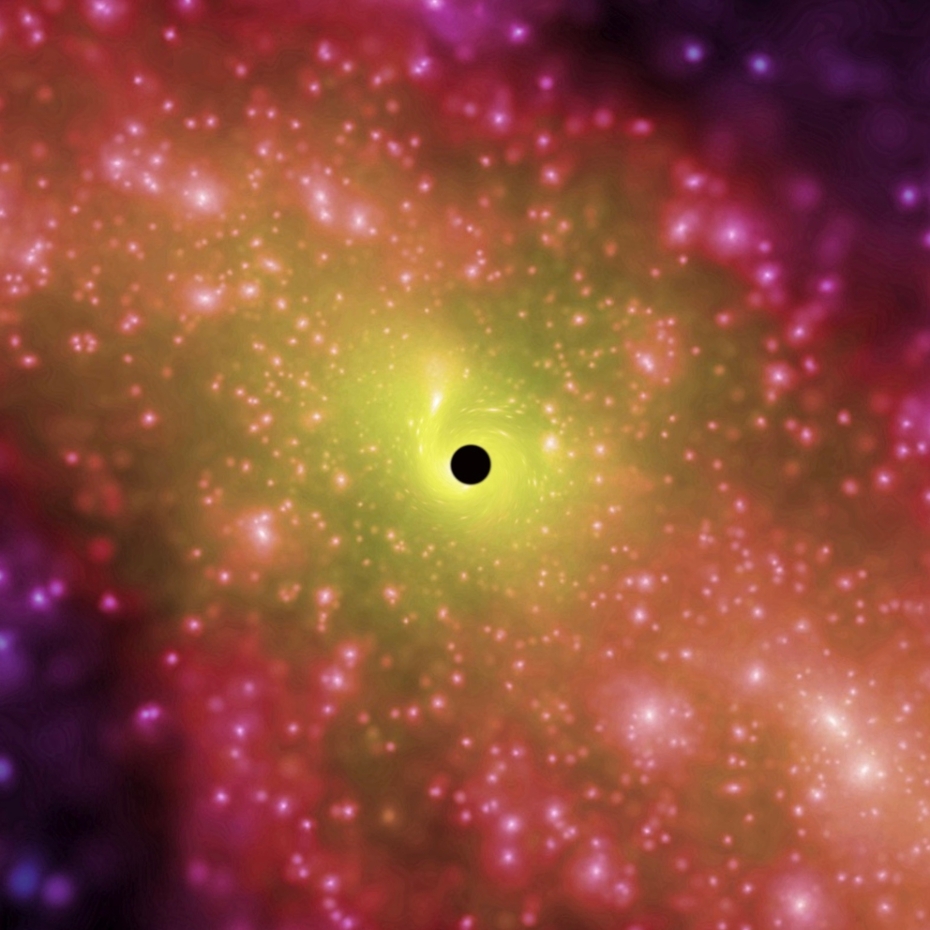
GRAPPA physicists Gianfranco Bertone and Samaya Nissanke receive NWO ENW-M2 grant for their research project "Searching for dark matter with gravitational waves"
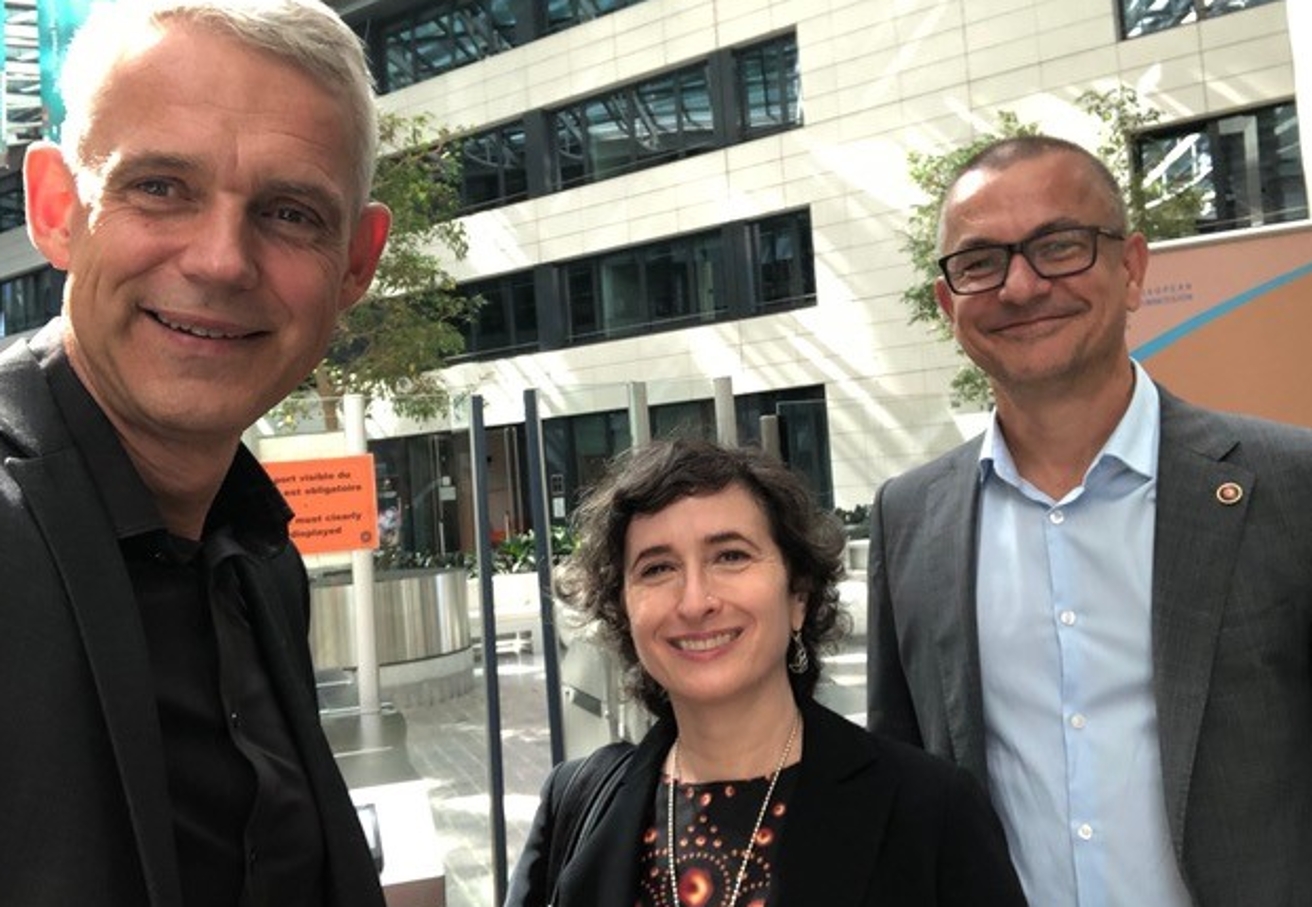
Astronomers receive ERC Synergy Grant to make colour movies of black holes and build new telescope in Africa
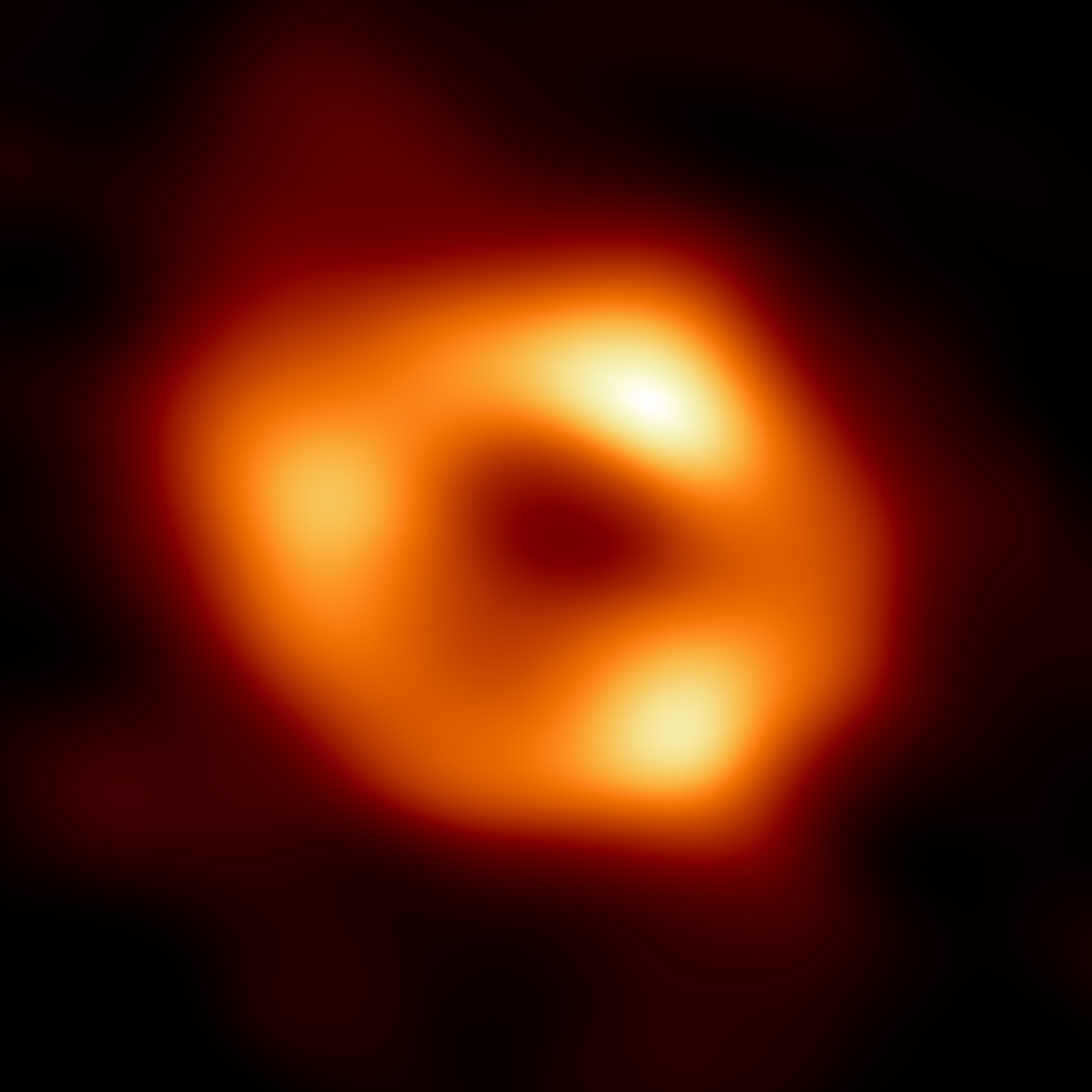
Astronomers Reveal First Image of the Black Hole at the Heart of Our Galaxy
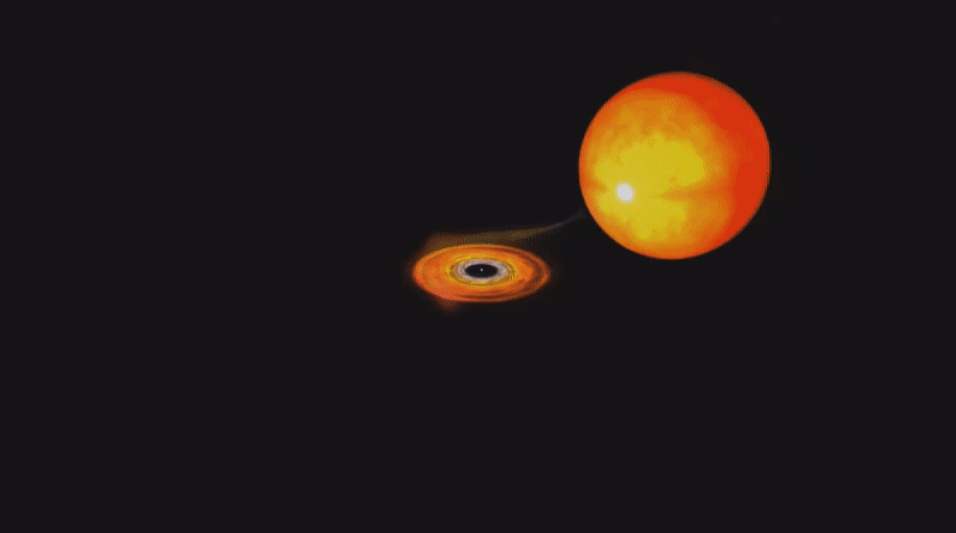
Millisecond Pulsars from Accretion Induced Collapse naturally explain the Galactic Center Gamma-ray Excess
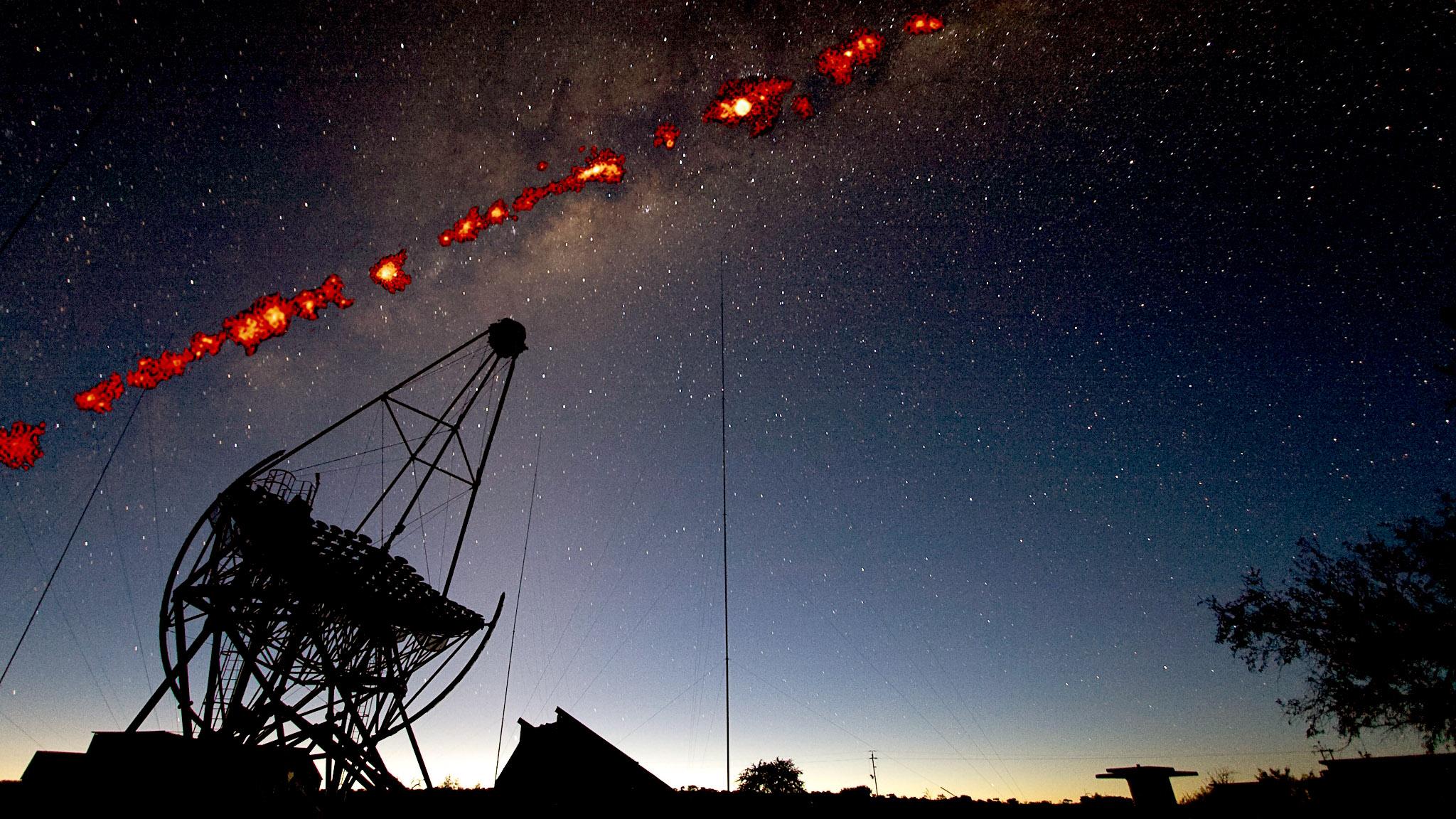
Jacco Vink to use large grant to seek out the sources of gamma rays
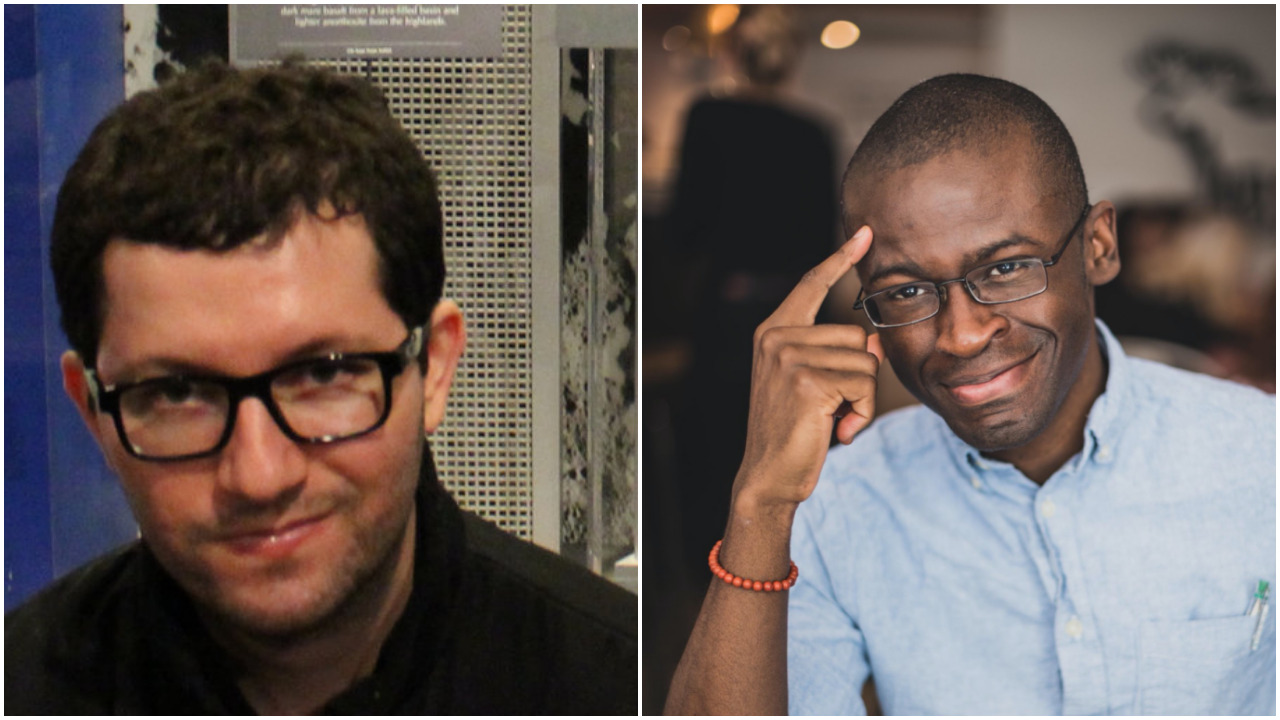
GRAPPA Postdoctoral Fellows: Lionel London and Oscar Macias
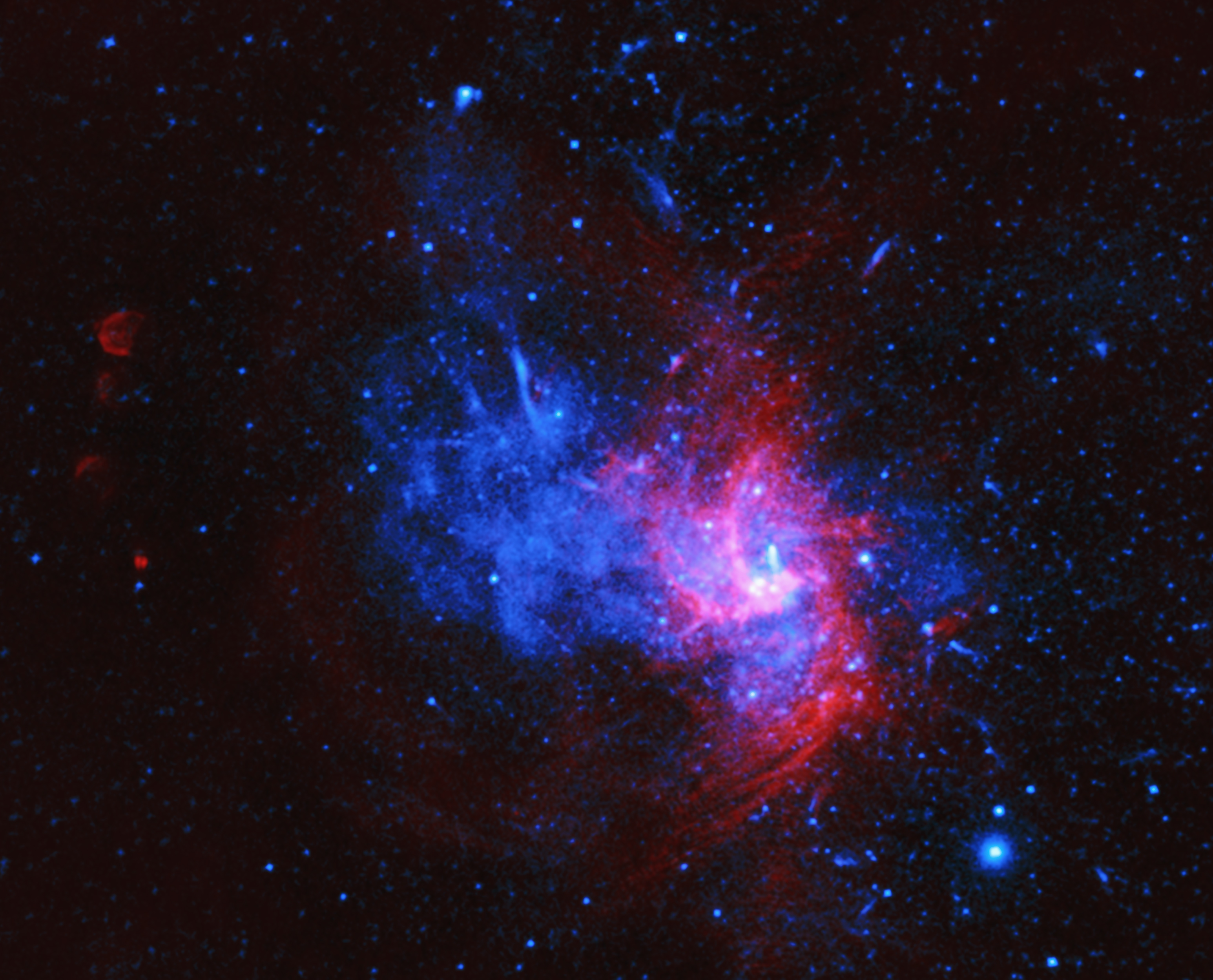
Rare Blast’s Remains Discovered in Milky Way Center
Opening of GRAPPA Postdoctoral Fellowship!
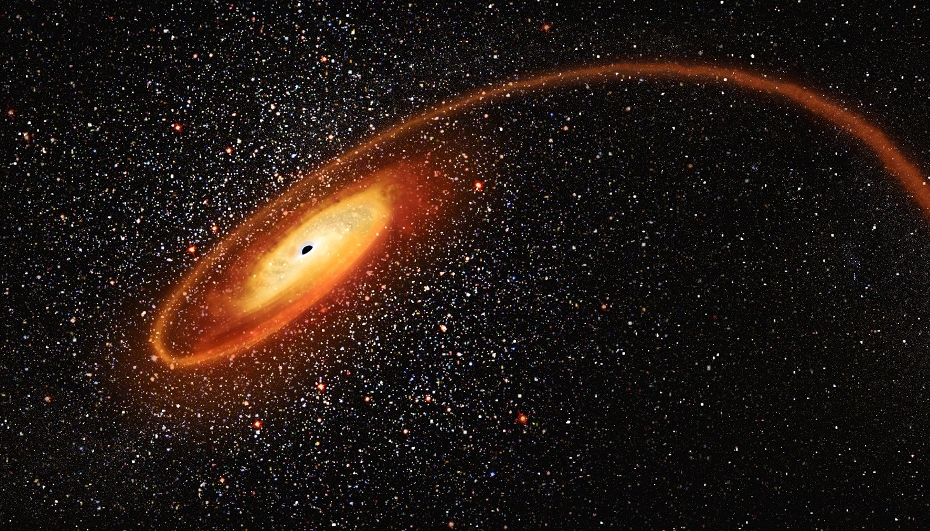
The Dutch Black Hole Consortium receives €4.9 million in funding
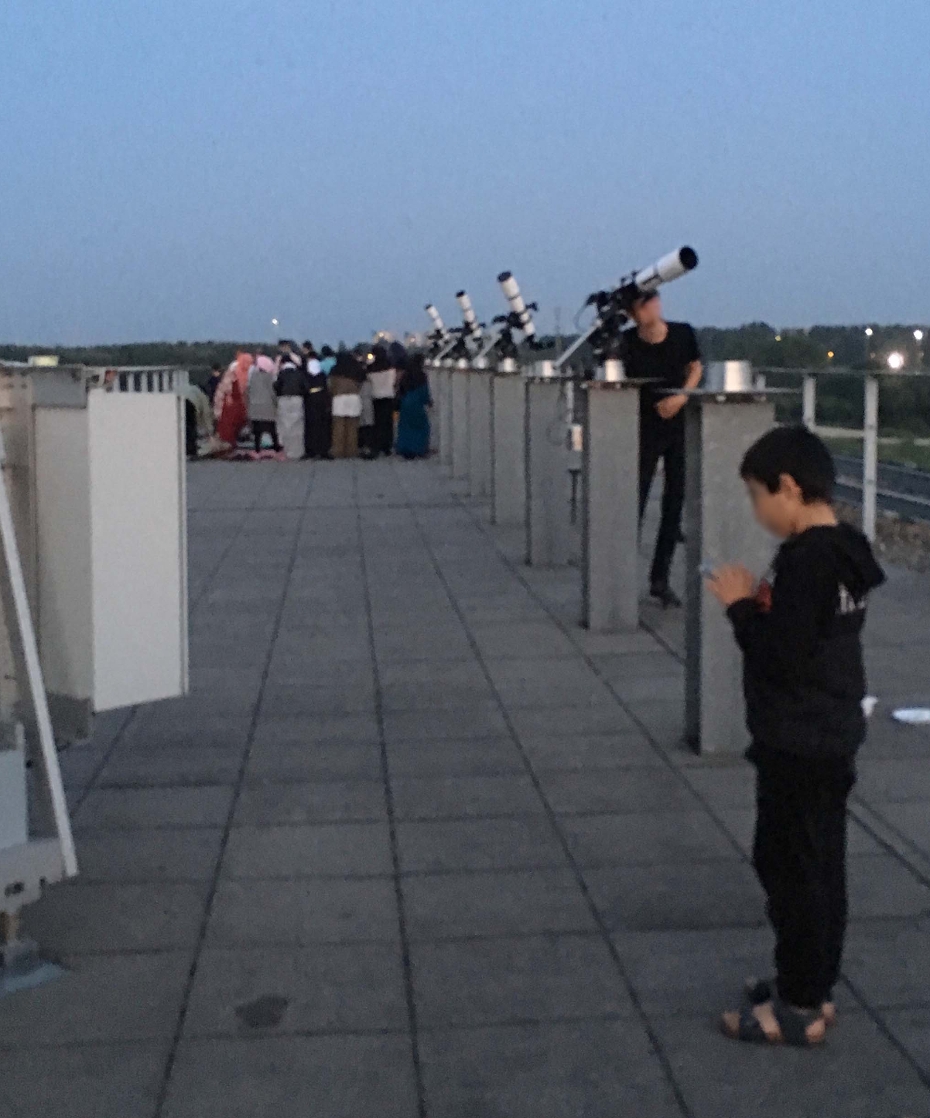
Sera Markoff receives Diversity Initiative Award
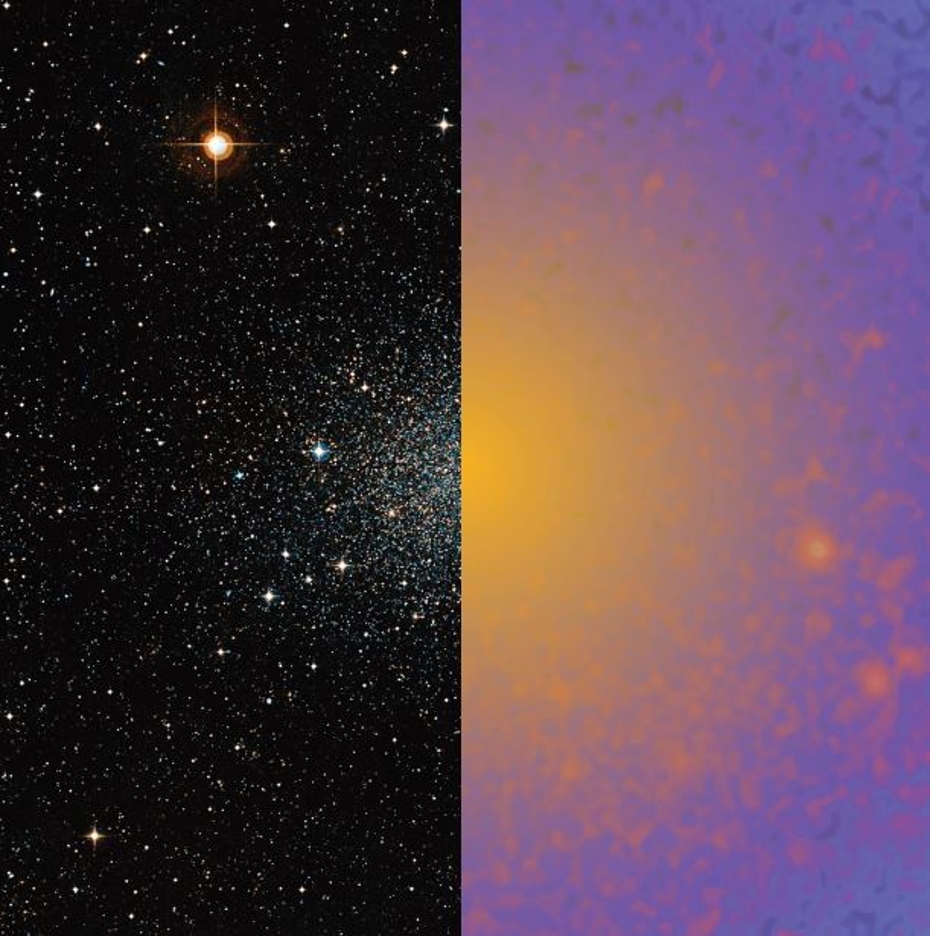
Dark matter even more elusive than previously thought
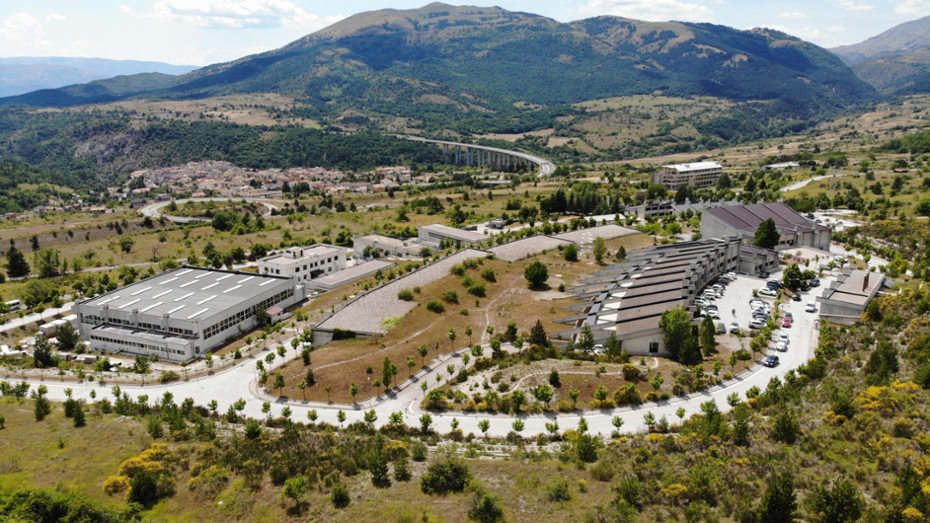
NWA-ORC for neutrino observations
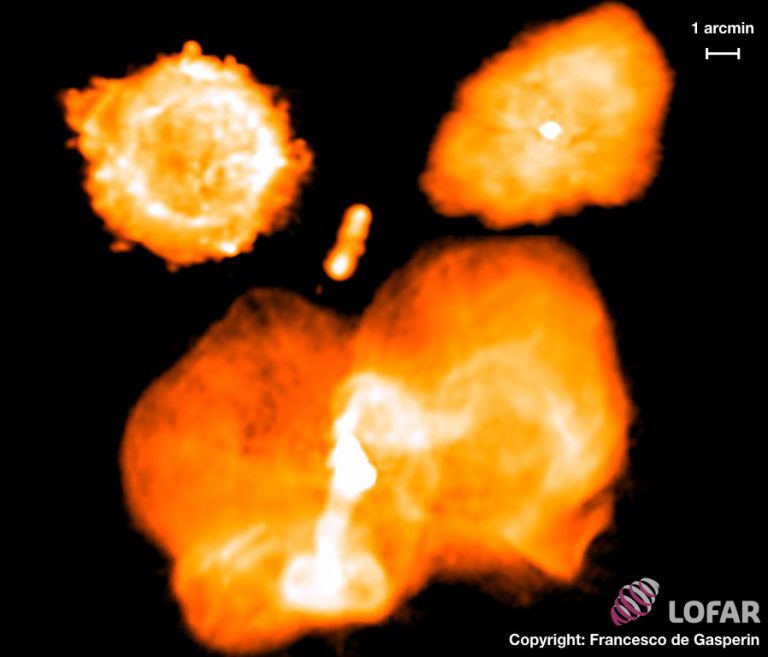
LOFAR images cosmic radio monsters
Top prize in high-energy awarded to Event Horizon Telescope
Archived News (pre-2019)
- Samaya Nissanke elected GRAPPA spokesperson
- Gianfranco Bertone appointed founding director of EuCAPT
- Kenny Ng to join GRAPPA on a Marie Curie Individual Fellowship
- Mass or interaction, but not both
- A new era in the quest for dark matter
- Another blow for the dark matter interpretation of the Galactic Center Excess
- Bachelor workshop results in publication on neutrino decay
- TeVPA 2018
- Welcome, Samaya Nissanke!